Electron Microscopical Reconstruction of the Anterior
Sensory Anatomy of the Nematode
Caenorhabditis elegans
Samuel Ward, Nichol Thomson, John G. White and Sydney Brenner
MRC Laboratory of Molecular Biology, Hills Road, Cambridge CB2 2QH
England and Department of Biological Chemistry, Harvard
Medical School, Boston, Massachusetts 02115 U.S.A.
Abstract -
Introduction -
Material & Methods -
Results -
Discussion -
Acknowledgments -
References
*New nomenclature equivalents for this paper*
Abstract
The complete structure of the anterior sensory nervous system of the small nematode C. elegans has been determined by reconstruction from serial section electronmicrographs. There are 58 neurons in the tip of the head. Fifty-two of these are arranged in sensilla. These include six inner labial sensilla, six outer labial sensilla, four cephalic sensilla and two amphids. Each sensillum consists of ciliated sensory neurons ending in a channel enclosed by two non-neuronal cells, the sheath and socket cells. The amphidial channel opens to the outside as does that of the inner labial sensilla so that these probably contain chemoreceptive neurons. The endings of the other sensilla are embedded in the cuticle and may be mechanoreceptive. The cell bodies of all the neurons lie near the nerve ring and their axons project into the ring or into ventral ganglia. One of the ciliated sensory neurons in each of the six inner labial sensilla makes direct chemical synapses onto a muscle making the sensory-motor neurons. The anatomy of four isogenic animals was compared in detail and found to be largely invariant. The anatomy of juveniles is nearly identical to that of the adult, but males have four additional neuron processes.
Introduction
Nematodes have simple nervous systems composed of only a few hundred neurons. Seventy years ago, R. Goldschmidt made a detailed study of the nervous system of Ascaris by reconstruction from serial sections using optical microscopy. (Goldschmidt, '03, '08, '09). Among his elegant anatomical drawings he produced a set of enigmatic wiring diagrams which have never been interpreted. It is likely that some of the bizarre connections shown in the diagrams were simply the outcome of the low resolution of the method Goldschmidt used. A smaller nematode would offer the possibility of determining the complete structure of a nervous system by electron microscopy allowing reliable identification of connections. This would open the way to understanding how the nematode's behavior could be generated by the nervous structure.
We have chosen the small soil nematode Caenorhabditis elegans for electron microscopical reconstruction of its nervous system. C. elegans is a favorable organism for studying the gentics of complex processes and many behavioral mutants have been isolated (Brenner, '73; Ward, '73; Brenner, '74). Reconstruction of the wild-type nervous system will make it possible to recognize anatomical lesions in such mutants. Such lesions should aid the task of understanding nervous system function by allowing correlation of behavioral and anatomical defects. In addition, the study of genetically induced lesions may help to understand how nervous system structure is specified gentically.
This paper is the first of a series which will describe the structure of the nervous system of C. elegans. It deals with the anterior sensory nervous system. Although, in volume, this is only a small part of the nematode it accounts for 58 of the 300 or so neurons found in the animal. The neurons are relatively simple and the reconstruction, though laborious, has not posed any special problems.
Materials & Methods
Organism
The nematode used in this work was Caenorhabditis elegans (var. Bristol). It was grown at room temperature on agar petri plates seeded with E. coli as described by Brenner ('74).
Fixation and embedding
Worms were rinsed off a fresh petri plate and fixed in 0.5% OsO4 in 0.1 M sodium phosphate, pH 7.4, for one hour at room temperature. Some specimens were prefixed in glutaraldehyde by rinsing them off plates with ice-cold 3% glutaraldehyde in 0.1 M sodium phosphate, pH 7.4, and then cutting them in half to allow penetration of the fixative. After two hours on ice, worms were removed from glutaraldehyde by centrifugation, rinsed and then post-fixed in OsO4 as above.
After fixation, the worms were spread on a thin layer of 1% agar and any uncut worms were cut in half or cut nearer the head if only the tip of the head were going to be sectioned. The cut worms were covered with a drop of 1% agar, and blocks of agar containing a single specimen were removed, dehydrated through a graded series of alcohols to propylene oxide, then propylene oxide and araldite (CY 212 resin, CIBA, Ltd.) and then into araldite at room temperature overnight. The following day they were transferred to fresh araldite and polymerized in gelatin capsules overnight at 60°C.
Sectioning, staining and electron microscopy
Serial sections of approximately 500 Å were cut with diamond knives on an LKB Ultratome III. Most of the series were cut transversely to the long axis of the nematode but several series were cut longitudinally. Ribbons of sections were routinely picked up on formvar-coated 75-mesh copper grids taking care not to align a ribbon with a grid bar. When every section was needed ribbons were collected on formvar-coated slot grids. For analysis of the sensory endings mesh grids were satisfactory. Grids were stained with 5% aqueous uranyl acetate for ten minutes at 60°C and then with lead citrate for five minutes at room temperature according to the procedure of Reynolds. Each section was photographed onto a single plate with an AEI EM 6B or AEI EM 802 electron microscope, except for the region of the nerve ring which was photographed in four parts and the prints assembled into a montage.
Reconstruction
The anterior tip of the head of the nematode contains the specialized endings of sensory neurons and processes of a number of associated cells. In this region the processes have distinctive morphological features which can be reconstructed from about 200 serial sections. Four such series have been analyzed completely, and many of the features have been confirmed in less complete series from about 25 additional animals. Each series of sections was enlarged and printed in rough alignment. All individual cell processes were followed from print to print and labelled with colored pens on successive photographs. Small groups of processes were followed together to make identification easier and to prevent accidental jumps.
The ciliated endings of many of the processes in the head suggested that these were dendrites of sensory neurons. To confirm this and to determine the axonal projections of these neurons, every process in the head was followed in its entirety. This involved complete reconstruction of a series of 1,600 sections which was carried out as follows. The processes were traced back from the tip of the head for 850 sections. They form six nerve cords each of which was traced independently. At the same time reconstruction was begun from the other end of the same series, identifying cell bodies and their axonal projections. When the two sets of assignments were joined, symmetrical cells were compared. Complete concordance was found. Thus the classification of cells by their positions and axonal projections matched the classification of processes by the similarity of their neuron endings. The central structures have been confirmed on an independent series but tracing the nerve cords has only been done once. Nevertheless, the concordance found gives us confidence in the assignments made.
The diagrams showing the structures of the various sensilla and the positions of cell bodies (figs. 4, 14, 18, 40) were prepared by tracing the outlines of cells in a sensillum from equally spaced transverse sections. The longitudinal projection of the structure was then drawn by hand, Longitudinal series of sections confirmed that interpretations based on transverse sections were correct.
To reconstruct the shapes of the amphidial cells the transverse outlines of each cell were traced into a computer directly from the photographs using a coordinate digitizer to draw the cell outlines. A computer graphics terminal then displayed longitudinal projections which could be rotated to aid visualization. The drawings of these cells shown in figures 19, 20 and 21 were prepared from print-outs of the computer display. The details of the system will be described elsewhere.
Terminology
We call all the sense organs in the tip of the nematode's head "sensilla" which cornforms with the definition given by Bullock and Horridge ('65, p. 1608) "...simple types of sense organs involving only a few neurons." In much of the earlier nematode literature, sensilla has been used only for the amphids; other sense organs are designated as "papillae, setae, etc." We also adopt two new terms "sheath" and "socket" cells for the two non-neuronal cells invariably associated with each sensillum. The reasons for this are presented in the discussion. We have followed the nomenclature of DeConinck ('65) in naming the individual sensilla.
Results
The arrangement of sensilla The tip of the head of C. elegans is shown en face in the scanning electron micrograph, figure 1. There are six symmetrical lips surrounding the triangular opening of the mouth. Each lip is surmounted by a papilla which is the ending of the inner labial sensillum. The lateral lips contain an inpocketing of the cuticle which is the opening of the amphid. Each sub-dorsal and sub-ventral lip has a pair of bumps and the lateral lip has a single bump visible. These are the endings of the cephalic and outer labial sensilla.
All of the cells found in the anterior 10 um of C. elegans head are summarized in table 1. The non-neuronal cells were identified easily by their positions and morphology. The neurons were identified by synaptic connections in the central nervous system. Most of the neurons can also be recognized just by the fine structure of their anterior terminals.
The types of neurons in the tip of the head are summarized in table 2. (The labels given in this table will be used throughout this paper.) There are a total of 58 neurons arranged in bilateral, or apparent four and six-fold symmetries. This symmetry reduces the types of neurons to 21. With two possible exceptions (see below) all of the neurons appear to be sensory. Figure 2 is a micrograph of a transverse section cut 1 um from the tip of the head which shows the arrangement of the sensilla. Each of the six lips contains one inner labial and one outer labial sensillum. The sub-dorsal and sub-ventral lips each contain a cephalic sensillum. The lateral lips each contain an amphid. This arrangement of sensilla is summarized by figure 3 which shows diagrammatically all the sensilla and all the neurons in the head. The structure of each of these sensilla will be presented in the following sections.
Figure 1. En face scanning electron micrograph. The six papillae surmounting the lips are the endings of the inner labial sensilla. Other sensilla endings are the small bumps and the inpocketing of the amphids which are labeled as indicated in table 2. This picture was taken by Mr. Len Christianson and Dr. Peter Andrews and kindly provided by Dr. David Hirsh. Magnification, x8,000.
Table 1: Cells in the anterior 10µm of C. elegans
Cell type |
Number |
Muscle |
8 |
Hypodermal |
8 |
Labial
Epidermal
Arcade |
6 9 |
Sheath |
18 |
Socket |
18 |
Neuron |
58 |
Total cells |
125 |
The hypodermal cells ring the circumference of the nematode and secrete the cuticle. The muscles lie longitudinally just under the hypodermis. The labial epidermal cells form the divisions of the six lips. The arcade cells line the esophagus: three syncytial anterior cells overlap six syncytial posterior cells. The nuclei of all the above cells lie 50-100 um from the tip of the head. The sheath, socket cells and the neurons make up the sensilla.
The inner labial sensilla
The structure of an inner labial sensilIum is diagrammed in figure 4. Electron micrographs of transverse sections of this sensillum are shown in figures 5-13, and a longitudinal section in figure 15. Two sensory neurons innervate each sensillum. The ending of inner labial neuron 2 penetrates through a small opening in the cuticle at the tip of a papilla (figs. 4, 5, 6). The other neuron ends embedded in the cuticle approximately 0.5 um below this opening (figs. 4, 7). Both neuron processes then pass back through a channel in the cuticle and sub-cuticle. This channel is lined by extra-cellular material and bends inward before being encased by a specialized non-neuronal cell, the socket cell. The socket cell wraps around the channel approximately 1 um from the ending and seals to itself with a tight junction (figs. 28-30 for similar junctions). The extracellular material lining the channel ends where the socket cell forms a tight junction to another specialized non-neuronal cell, the sheath cell.
The sheath cell is goblet-shaped and surrounds the nerve channel. The neurons penetrate through the base of the sheath cell to reach the inside of the channel with tight junctions sealing the channel from the pseudo-coelom. The sheath cell membrane (the inner surface of the goblet) is deeply invaginated into lamellae below the region where the neurons penetrate the sheath cell. The spaces between lamellae are continuous with the sensory neuron channel, as drawn in figure 4 and shown in figure 10, 11 and 12.
The endings of the two inner labial sensory neurons have characteristic fine structure. Sensory neuron 1 has a basal body with seven doublet microtubles. These extend various distances up the nerve in a ciliary ring, some reaching the tip and fusing with an electron dense material This neuron also has a striated ciliary rootlet extending posteriorly from its basal body for about 7 µm as shown in figure 15. There are one to four vesicles in this nerve just below its basal body. In glutaraldehyde fixed preparations the vesicles range in diameter from 250-900 Å and do not contain electron dense material.
Inner labial neuron 2 is exposed to the outside at its tip. It has a basal body with five to seven doublet-microtubules about 2.5 um. from its tip. These doublet tubules become singlets as they extend toward the tip, and two of them extend to the very end of the neuron. There is no ciliary rootlet.
Figure 4 shows an unciliated accessory neuron ending adjacent to a socket cell. A cell of this type is found invariably associated with the sub-ventral sensilla. In some animals this cell has a branch to the lateral sensilla as well. These accessory neurons sometimes end embedded in the socket cell but do not have specialized junctions at their endings. The dorsal sensilla have a similar neuron associated with them but this dorsal neuron is a branch of the lateral accessory neuron m discussed below.
The lateral inner labial sensillum has two additional ciliated accessory neuron labeled m and n. These neurons wrap around a branch of the lateral inner labial socket cell just below the region where the socket cell surrounds the neurons (fig. 2). Each contains a basal body near its ending containing nine doublet microtubules which extend anteriorly for about 1 um. The neuron m also has a short ciliary rootlet.
Fig. 2 Transverse section 1 um from the tip of the head. Sensilla and neurons are labeled as in table 2. The right sub-ventral side is more anterior than the left sub-dorsal. The specimen was prefixed in glutaraldehyde. Magnification, x 13,000
Fig. 3 Summary of the arrangement of neurons and sensilla in the head. All of the sensilla are shown as neurons surrounded by sheath cells (lightly shaded) with socket cells (darkly shaded) adjacent. Ciliatcd neurons are black, unciliated neurons are unshaded. Labeling is as in table 2.
Fig. 4 Inner labial sensillum. A right sub-ventral sensillum is shown diagrammatically in longitudinal and transverse section. The six inner labial sensilla have identical structure except for the accessory neuron. The outlines of the cells were simplified slightly from actual tracings and the number of membrane invagination in the sheath cell was reduced for clarity. The blackened junctions between cells represent tight junctions. Vesicles are shown near the basal body of neuron 1. The socket cell and the anterior part of the sheath cell are surrounded by a labial epidermal cell to which the socket cells make additional tight junctions. This cell is visible in figure 8 and 9.
Fig. 5,6 Tip of the inner labial sensillum. The very tip of the papilla is shown in transverse section, revealing that the inner labial neuron 2 opens to the outside through a channel in the cuticle. Figure 5 is a right sub-dorsal sensillum and figure 6 is a right sub-ventral sensillum from different animals. Magnification, x 21,000.
Fig. 7 Transverse section 0.5 um from the tip of an inner labial sensillum. This electronmicrograph shows the ending of the inner labial neuron 1. Magnification, x 21,000.
The outer labial and cephalic sensilla
The six outer labial and four cephalic sensilla end beneath the cuticle and have similar structures. The lateral pair of outer labial sensilla differ somewhat from the sub-dorsal and sub-ventral pairs. The sub-ventral cephalic and outer labial sensilla are shown diagrammatically in figure 14. Figure 8-13 are electron micrographs of transverse sections through these sensilla.
In each sensillum a single neuron passes through a close-fitting channel about 1.5 microns long through the cuticle and sub-cuticular matrix and ends embedded in the cuticle. The neuron channel widens slightly where the socket cell wraps around it and seals to itself. Like the inner labial socket cells, the socket cell abuts onto a sheath cell with a tight junction. The sheath cell is again goblet-shaped with the neuron penetrating into the channel through its base. The channel is sealed from the pseudocoelom with tight junctions between the neuron and the sheath cell. Both the outer labial and the cephalic sheath cells have deeply invaginated membrane lamellae separated by spaces which are continuous with the channel surrounding the neuron.
The cephalic and outer labial neurons differ from each other in size and in the fine structure of their tips. Each cephalic neuron has a basal body with six to eight doublet microtubules which extend toward the tip for about a micron in a ciliary ring. The neuron widens just above where it enters the subcuticular matrix, and its microtubules are joined by other tubules organized in groups around an amorphous electron dense core. These tubules extend to the end of the neuron. The neuron has no ciliary rootlet.
The sub-dorsal and sub-ventral outer labial neurons have basal bodies with five to seven doublet microtubules. Apparent singlet tubules extend anteriorly from the basal body in a characteristic joined diamond pattern, which reaches almost to the end of the neuron (fig. 10). A striated rootlet 3 um long extends posteriorly from the basal body of the sub-dorsal and sub-ventral neurons. Both the cephalic and outer labial neurons have a small electron dense branch which extends to a bump in the cuticle without penetrating through the cuticle. This bump is visible in the scanning elec- tron micrograph, figure 1.
The lateral outer labial sensilla have a neuron, sheath and socket cell arrangement similar to the sub-dorsal and sub-ventral sensilla, but the fine structure is different. The neurons end about 0.5 um less anteriorly than the other outer labial sensilla. Their endings are shorter and smaller and are electron dense. These endings resemble the small electron-dense bump of the other outer labial neurons without the anterior extension. Their basal body is within 1 um of the endings and does not have a striated rootlet connecting to it. Because of these differences in fine structure, the designation of these lateral sensilla as outer labial is based on other similarities to the sub-dorsal and sub-ventral sensilla: the positions of their cell bodies; the similarity of then arrangement in the nerve cords; and the position and morphology of their sheath cells.
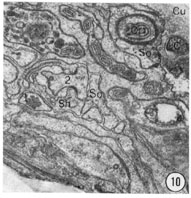
Fig. 8-13 Series of transverse sections through the inner labial, outer labial and cephalic sensilla. The series is through a right sub-dorsal lip of a single animal fixed only with osmium. Labeling is as in table 2 and in addition. So, socket: Sh, sheath: Cu, cuticle. The sections are approximately 0.5 um apart and correspond roughly to the transverse sections diagrammed in figure 4 and figure 14. Unlabeled processes are branches of non-neuronal cells or of the right amphid. Magnification, x 26,000.
Fig. 14 Outer Iabial and cephalic sensilla. The left sub-ventral outer labial and cephalic sensilla are shown in longitudinal and transverse section in a manner similar to figure 4.
Fig. 15 Longitudinal section of an inner labial sensillum. The section is cut longitudinally tangential to the cuticle. The electron-dense tip of the papilla is shown. Below that, the section crosses the subcuticular endings of the outer labial and cephalic sensilla. The junction between the socket and sheath cells is indicated by arrows. The striated ciliary rootlet in neuron 1 shows clearly. The average spacing of striations is 750 Å. Magnification, x 14,000.
Amphid General structure
The amphids are two large lateral sensilla each containing 12 sensory neurons and a sheath and socket cell. The amphid structure is shown diagrammatically in figure 18. Various aspects of the structure can be seen in figure 16-27 and figure 31 and figure 32. The general arrangement of neurons, sheath and socket cells shown in figure 18 is similar to that of the other sensilla. Eight of the neurons end in a channel which opens to the outside through the cuticle. This opening is formed by an infolding of the cuticle. The socket cell wraps around the channel and seals to itself as do the socket cells of the other sensilla. The socket cell is shown clearly in the longitudinal section, figure 17. The socket cell surrounds a sheath cell and joins to it with a tight junction (fig. 27). The neuron channel formed by the sheath cell is lined for part of its length by a densely staining extracellular material.
Sheath cell
The ending of the amphidial sheath cell is a large bilobed structure as shown in figure 19. The longitudinal section shown in figure 18 is drawn as if cut along the radial axis of the nematode parallel to the line of view of the sheath cell shown in figure 19. Figure 18 shows that the neurons enter the sheath cell at the base of its channel with tight junctions sealing their entry. Unlike the sheath cells of the other sensilla the amphidial sheath cell does not have deeply invaginated membrane lamellae opening into the neuron channel. Instead, it has a large golgi apparatus giving rise to many large vesicles along its concave face facing the channel (figs. 17, 18, 25).
Fig. 16 Tangential section through an amphid. This section shows the seam of the socket ceII particularly clearly, as well as clustering of the neuron endings (N) as they pass into the socket cell channel. Vesicles (v) inside thc sheath cell adjacent to the channel boundary are also evident. Magnification, x 14,000.
Fig. 17 Radial section through an amphid. The opening of the amphid through the cuticle is shown with the neurons passing up the amphidial channel. The large white-ish vesicles (v) on the right are inside the amphidial sheath cell. Magnification, x 14,000.
Neurons
Reconstructions of the endings of 12 sensory neurons which innervate the amphid are shown in figure 20 and 21. They all contain basal bodies with nine outer doublet microtubules located in the relative positions shown. They also contain 2-6 central singlet microtubules. In the endings e-l, the doublet tubules extend anteriorly in a ciliary ring nearly to the tip of each neuron and become singlet tubules near their endings (figs. 26, 27). Although the neuron endings e and g-k appear similar in morphology, each can be identified uniquely by the relative position of its basal body, its point of entry into the sheath cell, and, most importantly, its relative position in the sheath cell channel. This positioning will be described below. The eight cells e-l all pass up the amphidial channel nearly to its opening, as indicated in figure 18 and shown in figure 17. Because cells f and l are branched there are a total of ten ciliated processes passing up the amphidial channel.
The unusually shaped neuron endings a-d do not run all the way up the amphidial channel. Cells a-c run in the sheath cell channel up to their basal bodies but above this they poke their way into the sheath cell itself, like hands into a balloon, ending surrounded by the sheath cell. Cell d also pokes into the sheath cell but does so from outside of the sheath channel. It sends into the channel a small branch which is sealed off at its base by tight junctions to the sheath cell. None of these four neurons makes gap junction synapses to the sheath cell membrane; nevertheless, their shape creates an enormous surface area of membrane adjacent to the sheath cell membrane. There is always a 75-150 Å space between the two membranes. For cells a-c this space is continuous with the sheath cell channel. For cell d it is separated from the channel and the pseudo-coelom by tight junctions.
The arrangement of the four neurons (a-d) in the sheath cell is indicated in figure 18, 31 and 32. The cell with finger-like projections, d, is always dorsal; the branched cell a is dorso-lateral (it is the cell shown poking into the sheath in fig. 18); cell c has its wings extending both dorsally and ventrally; cell b has its wing ventral and the small branch dorsal in the channel.
Tracings of the cell positions at three different levels in the amphids are shown in figure 31; in figure 32 the arrangement of neurons in the left amphid of different adult animals is compared. The arrangement is identical in these four animals and is the same in males and juveniles. This invariant arrangement makes it possible to identify each amphidial neuron by its position in a single transverse section.
Other neurons
There are six neurons in the tip of the head which are not intimately associated with sensilla. Two of these neurons end in unciliated large bulbs, located between the sub-dorsal cephalic sheath cell and the amphidial sheath cell. These are labeled x in figure 3, 12 and 13. Four other unciliated neurons end in thin sheet-like structures. The ventral pair extends on the ventral side of the sub-ventral inner and outer labial sheath cells just below their anterior endings. The dorsal pair extends similarly between the dorsal inner and outer labial sheath cells. They are labeled y in figures 3, 12 and 13. These cells may be homologous to Goldschmidt's ('03) four "Faserzellen" in Ascaris.
Fig. 18 Amphid. A right amphid is shown in radial and transverse section in the manner of figures 4 and 14. The shapes of the cells were simplified slightly for clarity. Dense vesicles are shown near the basal bodies of the neurons. A large golgi apparatus and mitochondria are shown in the base of the sheath cell. The scale of the transverse sections is one half that shown for the longitudinal sections.
Fig. 19 Amphidial sheath cell. This drawing views a right amphidial sheath cell from the outside of a vertical nematode. The drawing was based on a computer reconstruction of cell outlines from series of transverse sections.
Fig. 20 Amphidial neurons a-d. The neurons are shown viewed from the side as for figure 19. They are based on computer reconstruction of transverse cell outlines. The rings of doublets indicate the position of the basal bodies.
Fig. 21 Amphidial neurons e-l. The neurons are shown as in figure 20.
Figs. 22-25 Transverse serial sections through an amphid. The series is through a right amphid of an animal fixed only with osmium. The neurons can be identified by comparison with figure 31. The sections are at approximately 1 um intervals. Magnification, x 18,000.
Figs. 26, 27 Fine structure of the amphidial neurons. High magnification electronmicrographs of transverse sections of the amphidial neurons of a glutaraldehyde-fixed specimen. (26) 3 um from the amphidial opening. Magnification, x 31,000. (27) 1 umm from the opening. Magnification, x 45,000.
Figs. 28, 29, 30 Cell junctions. High magnification pictures of the junctions between cells of a glutaraldehyde-fixed specimen. (30) A socket cell junction to itself. (29) A sheath-socket celljunction. (28) A sheath-epidermal cell junction. E, epidermal cell. Magnification, x 45,000.
Fig. 31 Arrangement of amphidial neurons. Tracings of transverse sections of the left and right amphids of a single animal are shown. The neurons are labeled as in figure 20 and 21.
Fig. 32 Comparison of the arrangements of amphidial neurons in different animals. The arrangement of neurons identified by their fine structure, shape and position is shown for three different animals. The left amphids are shown as in figure 31 at a level corresponding to the middle tracing in figure 31.
Males
Series of transverse serial sections through the tip of the head of three adult male worms have been examined. The arrangement of sensory cells is identical with that of the hermaphrodite except that there is an additional sensory process in the channel of each of the cephalic sensilla. This process is a modified cilium but is smaller than the cephalic nerve ending. It penetrates the cuticle of the male, opening to the outside through a small papilla as shown in figure 33. This opening is continuous with the cephalic nerve channel so that in the male the entire channel appears to open to the outside.
These four extra processes exit posteriorly from the sheath cell channel with tight junctions to the sheath cell, as do the cephalic neurons. It is not yet known whether they represent four additional neurons or are extra branches of neurons also found in the hermaphrodite.
Fig. 33 Male. The additional process which is in the male cephalic sensilla is shown ending exposed to the environment in the channel of an extra male papilla. This is a right sub-dorsal lip with the endings of the cephalic and outer labial neurons shown. Magnification, x 32,000.
Deirids
The deirids are a pair of lateral, cervical, sub-cuticular sensilla located about 70 um from the tip of the head. The ending of one of them is shown in figure 34. Like the other sensilla they have a ciliated sensory neuron in a channel surrounded by a socket cell and an invaginated sheath cell. The neuron channel does not penetrate the cuticle but ends longitudinally in the subcuticle, dorso-laterally. The fine structure of the ending closely resembles the endings of the cephalic sensilla showing many microtubules clustered around electron-dense material. Fluorescence microscopy of formaldehyde-treated worms shows that both the deirid and cephalic cell bodies and processes contain catecholamines and these six cells are the only anterior cells which do so (J. Sulston, personal communication).
Fig. 34 Deirid. The ending of the right deirid is shown. This transverse section was cut about 80 um from the tip of the head. The projections of the cuticle are the lateral "treads". D, deirid neuron. Magnification, x 27,000.
Juveniles
Figure 35 shows a complete section of a first or second stage juvenile. All of the sensilla are present and are identical with those in the adult hermaphrodite except that the sheath cells are not as extensive and none of the non-ciliated accessory neurons are branched.
Fig. 35 Juvenile. A complete transverse section about 0.5 um from the tip of the head of a juvenile is shown. Compare this figure to figure 2 and notice that the non-neuronal cells are much less extensive and that the sheath cells are smaller and less invaginated. Magnification, x 17,500.
Positions of cell bodies
The cell bodies for all cells in the anterior tip of C. elegans lie further back in the head. The neurons, sheath and socket cells connect to their cell bodies by long, thin processes that run down the head in six cords reflecting the arrangement of sensilla in the lips. Sections of the nerve cords are shown in figures 38 and 39. Although the sheath and socket cells run as nerve-like processes down the cords to their cell bodies, they do not give or receive synapses to any other cell and are, therefore, not neurons. In addition to the processes from cells in the tip of the head, 14 other processes are found in the anterior nerve cords.
These terminate about 15 um from the tip of the head, Two are interneurons which innervate the pharynx, and these are described in a subsequent section. Six others (one in each cord) are tiny processes from glial cells which line the inside of the nerve ring. Another four (2 sub-dorsal, 2 sub-ventral) are tiny processes from neurons which synapse onto muscles in the ring. Two more are tiny lateral processes from other neurons in the ring.
The positions of the cell bodies for all the processes in the anterior are shown in figure 40. Note that the positions of sheath and socket cell nuclei are not perfectly bilaterally symmetrical. The significant alterations are in the position relative to the first bulb of the pharynx. In the left dorsal cord, the outer labial socket cell nucleus is adjacent to the inner labial, anterior to the bulb, whereas in the right dorsal cord this outer labial socket cell nucleus is displaced posteriorly. A similar displacement is observed for the inner labial sheath cell in the two lateral cords. However, in all cases the sequence of cell bodies within each cord and the dorso-ventral positions of the cell bodies relative to the cords are the same. Two identical dorsal motor neuron cell bodies are also not bilaterally symmetrical: they are in reverse order on the two sides. The above variations in symmetry may be trivial due only to mechanical displacement by the pharynx so that the cell body positions actually reflect the bilateral symmetry of the sensilla in the head exactly.
The additional four- and six-fold arrangements of sensilla in the head are reflected in cell body position also but not as precisely. For example, if one compares the dorsal to the ventral cords one finds the same anterior-posterior sequence of neuron cell bodies (inner labial two, inner labial one, outter labial, cephalic) but the spacings and dorso-ventral displacements of the nuclei differ. The dorsal cephalic cell bodies are, in fact, posterior to the nerve ring itself. The positions of the lateral outer labial neuron cell bodies are similar to those of the outer labials in the dorsal and ventral cords and are not as posterior as the cephalic cell bodies, This is evidence supporting the designation of the lateral sensilla as part of the outer labial symmetry group.
Pharyngeal innervation
Two neurons found in the lateral cord enter the anterior end of the pharynx. This connection was described in Ascaris by Goldschmidt (Bullock, '65), and our attention was drawn to it in C. elegans by R. L. Russell (personal communication). Figures 36 and 37 show the extension of this neuron into the pharynx. The neuron ends in an electron-dense specialization adjacent to two pharyngeal neurons with a possible gap junction between them.
The two neurons entering the pharynx are interneurons which receive synaptic input in the ring and are afferent to the pharynx (White, Thomson and Brenner, unpublished). They are the only two neurons which connect the somatic nervous system to the pharynx.
Figs. 36, 37 Pharyngeal neuron. Figure 36 shows the pharyngeal neuron (IP) extending an electron-dense process into the pharynx. Figure 37 shows this process two sections later ending as a densely staining process with a possible gap junction to neuron (3) within the pharynx. The two neurons labeled 3 and 4 both may be associated with the ending of the pharyngeal neuron. They appear to be fused in figure 37, but successive sections show that this is not so: the membrane boundaries are not perpendicular to the plane of section figure 37. Both neurons 3 and 4 have cell bodies with the pharynx. Magnification, x 30,000.
Fig. 38 Sub-dorsal and sub-lateral anterior nerve cords. A transverse section approximately 30 u from the tip of the head. The sub-dorsal cord is the cluster of small processes at the top left. The amphidial neurons are the larger processes below the amphidial socket cell body; the small processes are from the other lateral sensilla and from other lateral cells (see tect). Magnification, x 13,000.
Fig 39 Neuronal cell bodies. A transverse section approximately 50 u from the tip of the head showing some of the neuron cell bodies on the right sub-dorsal side. mn motor neuron. Magnification, x 15,000.
Fig. 40 Anterior nerve cords and sensilla cell bodies. The nerve cords and cell bodies of all cells which have processes in the anterior nerve cords are shown. They are drawn as if projected radially onto a cylinder through the length of the worm and the cylinder were cut along the mid-dorsal line and unrolled. The positions of the first bulb of the pharynx and the nerve ring are noted on the side. In addition to previous labels, cells are labeled as follows: G, glia; mn, motor neuron; in, inter-neuron; P, pharyngeal neuron. Non-neuronal cells are shaded. Not all the cell bodies in this region are shown. The neurons changing cords in front of the pharynx bulb are m and I Ac.
Axonal projections
For clarity the axonal projections of the sensory neurons are not shown in figure 40. The axons of the sensory neurons, except for 11 of the amphidial neurons and neuron m, enter the circumpharyngeal nerve ring. The axons first pass posteriorly outside the nerve ring remaining grouped in symmetrical cords. They then turn back to form the neuropil of the ring making both gap junctions and chemical synapses en passant as they course anteriorly. Eleven amphidial neuron axons enter the ventral ganglia through commissures passing ventrally between the muscles and hypodermis. The synaptic connections of all of the neurons from the head have been determined. With the exception of the pharyngeal neurons and the neurons x, all the neurons give synapses in the ring, consistent with their designation as sensory. The axonal processes from all six inner labial neurons 1 make direct chemical synapses to muscle arms from the thirty-two most anterior body muscle cells. One such synapse is shown in figure 41. Each neuron makes several such synapses. This synaptic connection to a muscle makes the inner labial neuron a direct sensory-motor neuron. However, these neurons also synapse onto interneurons, including the neuron which connects to the pharynx, and they have a peculiar synapse-like association with hypodermal cells. The details of these connections and the synapses of the other neurons will be described elsewhere.
Fig. 41 Nerve ring. A transverse section of the right lateral and ventral regions of the nerve ring. The small processes labeled 1 and 2 to the lower left of center are the sub-ventral inner labial neurons as they pass posteriorly. After turning around they become larger and are the processes with vesicles labeled I-1 and I-2 which are passing anteriorly. The inner labial neuron 1 is also labeled at the top of the section. the arrow shows a synapse to a muscle process (M). The muscles in nematodes receive innervation by sending processes to the nerves; the process labeled M is from the most anterior head muscle. The synapse is recognized by its characteristic electron dense presynaptic specialization. It extends for several sections and this neuron makes four such synapses to the muscle. Magnification, x 17,000.
Discussion
Function
C. elegans has at least three sensory responses: chemical, mechanical and thermal (Ward, '73; Dusenberry, '73; Ward, unpublished). The chemical sense includes detection of at least four classes of attractants: cyclic nucleotides, anions, cations and hydroxyl ions (Ward, '73). The nematode is also repelled by acid, some form of carbonate ions, and aromatic compounds (Dusenberry, '74; Brenner and Ward, unpublished). From the altered chemotaxis of head-defective mutants, Ward ('73) concluded that the receptors detecting the attractants must be located on the head.
The structures of the sensilla on the head suggest which of them could detect these attractants, Chemoreceptive neurons must either directly contact the environment surrounding the nematode or contact another specialized cell which then contacts the surround. All of the neurons in the amphid meet these criteria, as does the inner labial neuron 2. Eight of the amphidial neurons, e-l, reach nearly to the opening in the cuticle of the amphidial channel. The three neurons a-c also contact the open channel further down. The neuron d has only a small branch reaching the open channel; however, it has a large surface area adja- cent to the sheath cell which bounds the channel, and therefore it might be a secondary sensory neuron such as is found in the mammalian taste bud (e.g., Murray and Murray, '70).
Amphids in other nematodes have been assumed to be chemoreceptors because they open to the outside, but no more direct evidence of their function has been presented (deConinck, '65; Bird, '71; Croll, '70). How- ever, the recent isolation of non-chemotactic C. elegans mutants which are defective in their amphidial neurons supports the interpretation that the amphid is a chemoreceptor (J. Lewis, personal communication).
The function of the amphidial sheath cell remains unknown. The large golgi apparatus with its forming face facing the amphidial channel suggests that it secretes material into the channel (Revel, '71). Presumably this material is stored in the large sheath cell vesicles and released into the channel, The sheath cell has been identified as a gland cell in other nematodes and esterase activities have been detected in the amphidial channel (Bird, '71; McLaren, '72). Its secretions may include mucus to protect the exposed neuron terminals or secretions which might be involved directly in neuron specificity or function.
The sheath cells surrounding all of the sensilla except the amphids have striking lamellar membrane invaginations which increase enormously the surface area of sheath cell membrane in contact with the neuron channels. Such membrane specialization might be for ion uptake and secretion so that these cells could regulate the ionic environment surrounding the sensory neurons thus affecting their sensitivity. The role of the socket cells appears to be support, but they may secrete the extracellular material lining the neuron channels.
The four additional sensory processes in the male cephalic sensilla are likely to be chemoreceptive because they contact the outside directly. A likely function for such a neuron would be to detect a sexual attractant released by a hermaphrodite. Sexual attractants have been described for several dioecious nematode species (Greet, '64; Green, '66; Cheng and Samoiloff, '71) but no evidence for asexual attractant in C. eleganshas been reported yet.
The mechanical and temperature sensitivity of C. elegans has not been studied extensively, but one can observe easily that a worm touched on the head backs up. Since we do not know how mechanical deformations are propagated along the cuticle we cannot predict which sensilla mediate this response. The inner labial, outer labial and cephalic sensilla are all candidates for mechanoreceptors because they have neurons ending embedded in the cuticle. They might also function as proprioreceptors since the fine structure of the cephalic sensilla resembles that of the proprioreceptive campaniform sensilla in insects (Moran et al., '72). Isolation of mutants altered in mechanosensitivity and identification of their anatomical defects might help to specify the function of these sensilla.
Comparison to nematodes and other invertebrates
The arrangement of nematode sensilla has been studied extensively with the light microscope because of its taxonomic importance (Chitwood and Wehr, '34; Chitwood and Chitwood, '38; DeConinck, '65). Chitwood and Wehr proposed that the primitive ancestral pattern of labial sense organs should consist of three sensilla per lip plus the amphids. They could arrange the phylogeny of various species so that it reflected modifications of this basic plan. They noted with chagrin, however (and this is confirmed by DeConinck ('65)), that no nematode has been discovered with three sensilla (excluding the amphids) on its lateral lips. Because the dorso-lateral sensilla is invariably absent, DeConinck proposed a different symmetry arrangement for the ancestral nematode: an inner ring of six; one outer ring of six; and an outer ring of four sensilla plus the two amphids. DeConinck proposed that the outer ring of four reflected the bilateral symmetry of the body rather than the hexaradiate symmetry of the head.
The arrangement of cephalic sensilla in the head of C. elegans conforms to the general nematode plan with no dorso-lateral sensillum. However, the cervical deirid is located dorso-laterally; it is similar in fine structure to the cephalic sensilla and like the cephalic neurons the deirid neurons contain catecholamines. Therefore, the deirid could be regarded as homologous to the cephalic sensilla. Thus, these sensilla would have a hexaradiate arrangement, with the lateral member displaced, supporting the model proposed by Chitwood and Wehr.
The structure of individual sensilla in C. elegans resembles sensilla in other nematodes, Goldschmidt ('03) first described that two accessory cells were associated with most of the sensilla. Goldschmidt found only one associated with the amphid but this was later corrected by Hoepli ('25). The resolution of the electron microscope has allowed us to describe the topology of these cells more accurately and we have renamed the cell which corresponds to Goldschmidt's Geleitezelle (accompanying or escort cell) the socket cell, and the cell corresponding to the Stützelle (support cell) the sheath cell. One reason for introducing this new terminology is that Goldschmidt's missing the Geleitezelle for the amphid has caused a subsequent confusion in terminology for the two cells found associated with amphids in other nematodes. In addition the terms "accompanying" and "support" cells have been used differently by different authors and some reviewers have confused them with labial cells.
Ciliated endings of nematode sensory neurons have been described for several species (for references, see McLaren, '72) and the complete structure of amphids from electron micrographs has been described recently by McLaren ('70) for Dipetalonema viteae, and by Storch and Riemann ('73) for Tobrilus aberrans. The amphid in D. viteae is similar in structure to that of C. elegans and has accessory cells corresponding to the sheath and socket cells. The accessory cells appear to be present in T. aberrans as well from the electronmicrographs shown, but they are not described as part of the amphid. In both cases, neurons with modified cilia pass out through a channel open to the outside. Other neurons ending in the sheath cell were not described but are found in other nematodes (Burr and Webster, '71).
As has been noted before (Lee, '65), the sensilla in nematodes resemble those in other invertebrates, especially arthropods (e.g. Slifter, '70; Hayes, '71; Harris and Mill, '73). They all have ciliated sensory neurons surrounded by specialized accessory cells. This similarity probably reflects evolution of convergent solutions to the problem of how to get a sensory neuron into or through an exoskeleton. Nonetheless, the possibility that the similarities in structure reflect a common primitive invertebrate ancestor with specialized sense organs should not be automatically dismissed.
The sensory-motor neuron
The finding that all six of the inner labial neurons 1 make direct chemical synapses to muscle arms establishes that these cells are sensory-motor neurons. Direct sensory-motor neurons have been postulated as intermediates in the evolution of nervous systems. Coggeshall ('71) described a possible sensory-motor neuron in the sea hare Aplysia, and a possible sensory-secretory neuron has been described in Trichuroid nematodes (Wright and Chan, '73). Interestingly, Goldschmidt ('08) proposed that one of the anterior sensory neurons in Ascarismight make a direct neuromuscular connection, but he could not be certain of this connectivity using only the light microscope. Our results suggest that Goldschmidt was correct so that sensory-motor cells may be common in nematodes. Since the nematode is a primitive invertebrate, such cells may indeed represent an important stage of the evolution of complex nervous systems.
Invariance and symmetry
The detailed comparison of the sensory terminals of several worms reveals little anatomical variation. The shape and position of the ciliated neuron endings and the arrangement of sheath and socket cells were identical in all animals examined. This invariance is also reflected by the symmetry of the sensilla, The animals are exactly bilaterally symmetric. The striking hexaradiate symmetry is not exact. The lateral pair of each type of sensilla are distinct from the sub-dorsal and sub-ventral pairs: the outer labial have smaller terminals, the inner labial have additional accessory neurons, the "lateral cephalics," the deirids, are displaced caudally. In addition, the inner labial sensilla do not have a medio-lateral plane of symmetry because in all sensilla the neuron 1 is most dorsal. These variations might indicate the influence of position on the development of sensilla specified identically genetically.
Four of the accessory neurons (the ventral inner labial accessory and the neuron m) vary both between and within animals in the number and length of their terminal branches. However, since the branches are absent in juvenile animals the growth of these processes may be age dependent and the variations found to be due to slight differences in age of the animals sectioned. There is also slight variation in the fine structure within the terminals of some of the neurons: the number of doublet microtubules in the neurons of the inner, outer and cephalic sensilla is variable and the presence of vesicles in the region of the basal bodies varies from animal to animal. This variation probably reflects the "noise level" in the developmental pathways specifying these structures. Since the animals were grown under identical conditions and are likely to be isogenic (Brenner, '74).
Although individual worms are not precise replicas of each other down to the finest details they are remarkably exact copies. By a combination of properties each individual cell and its processes may be uniquely identified in different animals. This is clearly essential for the ultimate interpretation of the anatomical findings in terms of function, but it is also important because it allows small anatomical changes to be reliably recognized in sensory mutants without complete reconstruction. A number of non-chemotactic mutants have alrcady been isolated (J. Lewis, personal communication and S. Ward and P. St. John, unpublished) and other sensory-defective phenotypes are being sought. These will be useful to further probe the structure and function of the nematode's sensory nervous system.
Acknowledgments
We thank Mrs. Eileen Southgate and Mr. Paul St. John for their patient and painstaking help following processes through series of sections, and Mrs. Annette Lenton and Mr. Lazlo Mesaly for preparation of illustrations. Mrs. Susan Baker helped with the electron microscopy. This research was supported in part by a Special Fellowship to S. W. from the National Institute of Neurological Diseases and Stroke and by Grant GB 36970 to S. W. from the National Science Foundation.
References
|